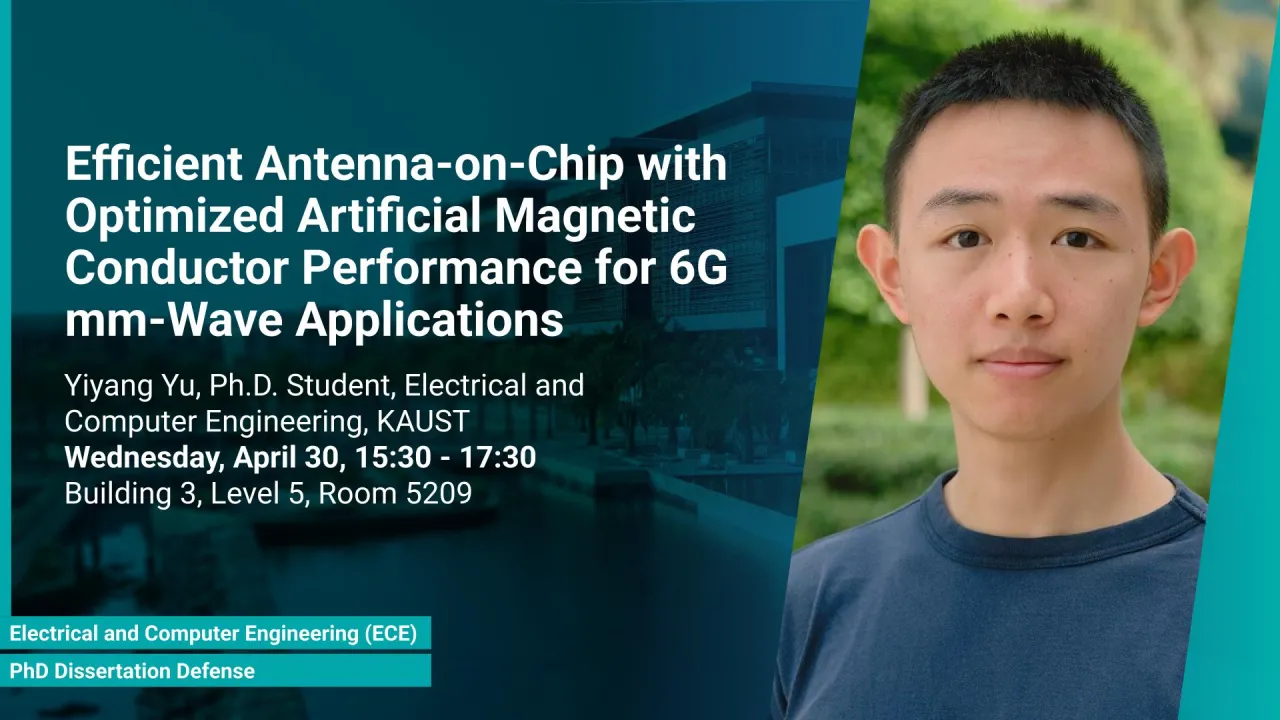
Efficient Antenna-on-Chip with Optimized Artificial Magnetic Conductor Performance for 6G mm-Wave Applications
This dissertation introduces innovative Artificial Magnetic Conductor structures and a reconfigurable superstrate to overcome profile thickness, illumination efficiency, and radiation pattern limitations in CMOS-integrated Antennas-on-Chip, thereby enhancing their performance and versatility for future 6G wireless communication systems.
Overview
The progress in wireless communication technologies is quickly moving the industry towards the sixth generation (6G) of networks. This new generation promises major improvements in data speed, reliability, response time, and the ability to handle advanced applications. 6G technology is exploring the upper millimeter-wave (mm-wave) spectrum, especially the 90–110 GHz range, which has been seen as a promising spectrum for development.
As operating frequencies increase, wavelengths decrease, leading to smaller antenna sizes. At the W band frequencies, antennas can decrease to just a few millimeters, making them compatible with typical chip dimensions and suitable for integration directly onto chips as Antennas-on-Chip (AoC). However, the radiation of typical AoCs is very poor because of the highly conductive silicon substrate.
A potential solution involves using the metal layers in the back-end-of-line (BEOL) stack-up of the Complementary Metal Oxide Semiconductor (CMOS) processes to create an artificial magnetic conductor (AMC) between the AoC and the lossy silicon substrate. The AMC isolates the AoC from the lossy substrate and provides in-phase reflection constructively, enhancing the AoC's efficiency. However, the implementation of an on-chip AMC presents several challenges: the AMC's thickness typically exceeds the limits of the ultra-thin stack-up of standard CMOS processes; the illumination across the AMC surface is uneven, particularly for the unit cells farther from the AoC; and the conventional AMC-backed AoC configurations yield broadside radiation patterns and horizontal polarization, which are unsuitable for certain applications requiring endfire radiation and vertical polarization (VP).
To address these challenges, the dissertation first proposes two innovative thickness reduction structures - Metallic Post (MP) and Embedded Guiding Structure (EGS) - that successfully reduce the AMC's profile by up to 41%, making it compatible with the ultra-thin CMOS stack-up. This design is realized through an in-house CMOS-compatible process that avoids traditional low-conductivity adhesion layers, thereby preserving the AMC's optimal in-phase reflection. Experimental results from a prototype demonstrate a significant gain improvement of 9.15 dB, with a measured gain of 5.85 dBi and a radiation efficiency of 57% at 94 GHz.
The second part of this thesis deals with the issue of ineffective illumination by introducing electric-field based coupling enhancement structures (E-CES) and magnetic-field based structures with vias (H-CES-V) or small metallic patches (H-CES-M), which boost the gain by 3.5 dB, 1.5 dB and 4.5 dB compared to conventional AMC, respectively. The prototypes of AMC-backed AoC with E-CES and H-CES-M demonstrate a boresight gain of 9.8 dBi and 10.4 dBi respectively.
The third and final part of this dissertation looks at adding frequency reconfigurability and versatility in achievable radiation patterns for AMC-backed AoC designs. This is done through the use of a multifunctional Alumina (Al2O3) superstrate with phase change material Vanadium Dioxide (VO2) thin film structures which provide the reconfigurability. This setup not only achieves vertical polarization and directional endfire radiation but also enables the switching between 94 GHz and 106 GHz - potential bands in the 6G spectrum - achieving gains of 5.2 dBi and 6.8 dBi, respectively. Additionally, the Al2O3 superstrate also serves as a protective cover (kind of package) for the chip.
In conclusion, this work not only addresses the inherent challenges of AMC-backed AoC designs but also advances the AoC field toward achieving the full potential of next-generation communication. The innovative solutions in this dissertation lay the groundwork for more compact, efficient and versatile wireless technologies.
Presenters
Brief Biography
Yiyang Yu is a Ph.D. Candidate at Integrated Microwaves Packaging Antennas & Circuit Technology Research Group under the supervision of Professor Atif Shamim at King Abdullah University of Science and Technology (KAUST). His current research interests include on-chip antenna design and artificial magnetic conduction design for 6G mm-wave applications.