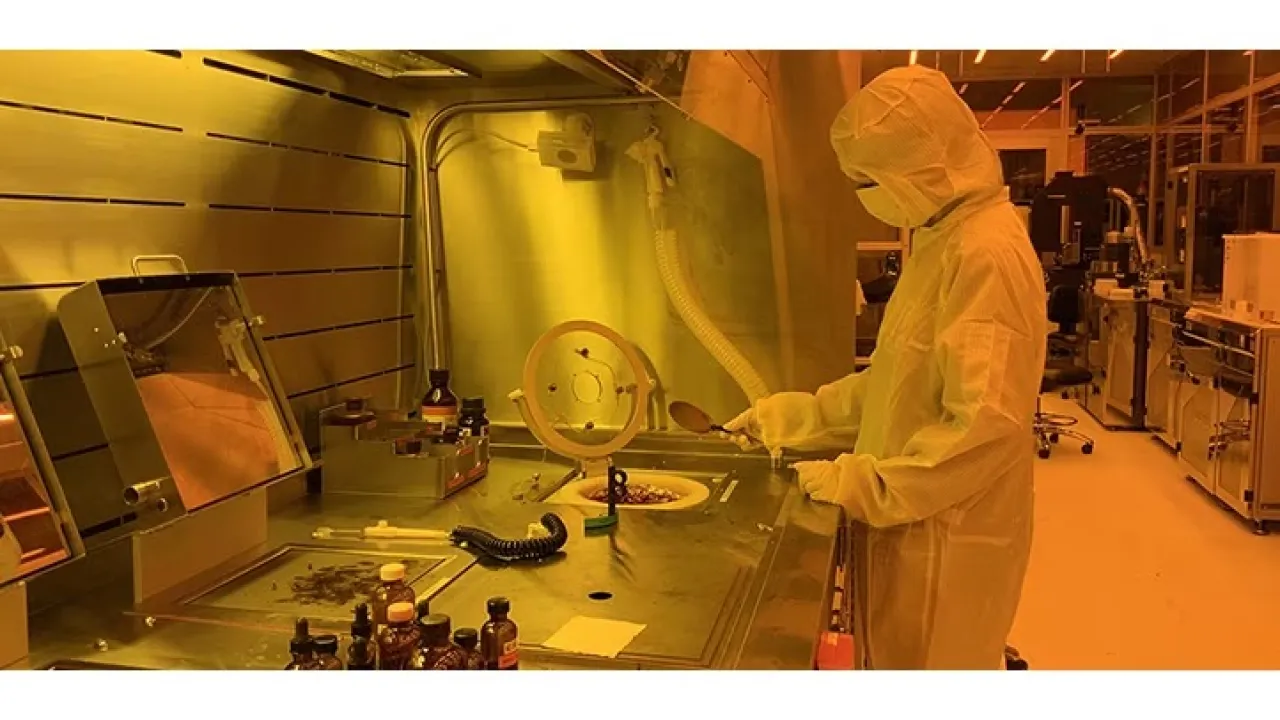
Flexing for success from Saudi Arabia to Silicon Valley
Asrar Damdam is setting up her own biotech company in Silicon Valley while pursuing a Ph.D. at KAUST
About
Asrar Damdam is proving that nothing can stand in her way. The 26-year-old KAUST Ph.D. student has already set up her own biotech company in Silicon Valley, and she is drawing attention from key investors there. She has published her master’s thesis as a pioneering concept on reconfigurable electronics as a featured article selected by a prestigious physics journal.
The Saudi Arabian scientist graduated from Effat University in Jeddah with a bachelor’s degree in electrical and computer engineering. Her main focus was on robotics and control systems, with a minor in photonics. A summer internship with Professor Muhammad Mustafa Hussain convinced her to stay at KAUST to pursue her master’s degree.
And that’s where her work took off.
Damdam worked on futuristic flexible electronics. Her master’s thesis was designing a silicon-based electronics platform that can change its shape and stretch in a variety of directions. “That’s what distinguishes my platform from others,” says Damdam. “Its capabilities go beyond flexibility and bi-axial stretchability. It can assume different shapes through multi-axial stretching.”
The details of her platform’s design and functionality were published in the journal Applied Physics Letters. “It’s highly prestigious for any research group to have a study published in this journal as a featured article, and our group is humbled by having the honor twice,” says Hussain.
Much research into flexible electronics has focused on using organic-polymer composites, due to their excellent mechanical properties and cost-effectiveness. Their downside is that their electronic properties cannot compare to those of silicon-based electronics. While silicon is an excellent, thermally stable semiconductor with high mechanical integrity, it is also rigid, fragile and cannot tolerate much strain.
Damdam set out to resolve this disparity using a "honeycomb-serpentine silicon platform." It is made from amorphous silicon and formed of multiple, connected honeycomb-shaped cells. The sides of the cells are made from six stretchable, snake-like "interconnects." Four more interconnects meet in the center of each hexagon at a circular disc that hosts the electronic components of the platform.
Damdam and her colleagues first designed a miniature 15-micrometer-thick platform that involved depositing onto a wafer several layers of materials, including amorphous silicon. The layers were each etched away to leave the patterned honeycomb-shaped silicon platform lying on top of the wafer. To release it from the wafer, Damdam used highly concentrated liquid hydrofluoric acid, leaving the tiny honeycomb-shaped silicon platform floating in the acid. “Removing the structures from the acid and then rinsing them was the most difficult part,” says Damdam. “I broke a lot at first, but after many failed attempts, I became an expert at removing them.”
“She never gives up,” says Hussain. “She keeps trying to address the challenges until they are resolved. A fusion of skills, experiences and a resolute mindset has been helping her to succeed.”
Damdam tested the stretchability of her microsized design and found areas for improvement. Following modifications, she designed a larger 2.5 x 1.8 centimeter-sized structure. Depending on how the structure was pulled, it could assume an irregular, star or quatrefoil shape. Its maximum stretchability was 14.2 percent beyond the original shape, but Damdam says this can be further improved by using ultrastretchable fractal interconnects. For comparison, human skin can be stretched up to 20 percent. Damdam says this means her design has potential for integration into wearable stretchable electronics devices and in soft robotics.
Read the full article